We spoke with Dr. Aaron Phillips about his Nature publications concerning artificially correcting hemodynamic instability following spinal cord injury, and how Kaha Telemetry helped him characterize cardiovascular regulation across multiple animal models.
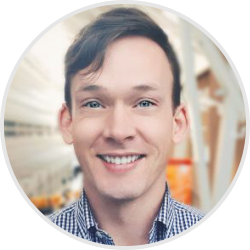
Dr. Aaron Phillips is an Associate Professor and Director of the RESTORE.network at the University of Calgary. Driven by his passion for the complex interactions between the nervous and cardiovascular systems, including anomalous interactions contributing to clinical disorders, Aaron's research focuses on developing novel therapeutics for people with neurological health issues.
In this article, we break down the development of the neuroprosthetic baroreflex, a device designed to artificially correct blood pressure after spinal cord injury, and the role the Kaha SNA and Pressure Telemeter played in characterizing cardiovascular regulation across multiple animal models. Aaron walks us through his Nature publications: Neuroprosthetic baroreflex controls haemodynamics after spinal cord injury, and Longitudinal interrogation of sympathetic neural circuits and hemodynamics in preclinical models which he co-led with Associate Professor Gregoire Courtine.
The debilitating effects of traumatic spinal cord injury are widely known. In addition to medication and rehabilitation, new treatment technologies explore electrical stimulation of damaged nerve centers to rescue muscle control. The Phillips Lab uses this technology to address a lesser-known symptom of spinal cord injury - hemodynamic instability. Designing a sophisticated device to electrically stimulate the nerve centers responsible for maintaining life-threatening unstable blood pressure.
“Cardiovascular instability after spinal cord injury is really broken into two phases,” Aaron says. “There is the acute stage in those first 24 hours after injury, and then the chronic stage. During that acute stage, there is something called neurogenic shock, and it's a massive depression of the sympathetic nervous system, very low blood pressure. It prevents the healing of the spinal cord. It directly relates to neurological damage. Roughly fifty percent of the injury is after the initial trauma, during neurogenic shock, when there is insufficient blood flow to heal the spinal cord.”
Hemodynamic instability, the inability of the body to maintain consistent blood flow and pressure, occurs during the chronic stage of spinal cord injury.
This instability leads to repeated hypotensive episodes; increasing the risk of stroke and heart disease, while seriously impacting recovery from spinal cord injury. Although pharmacological treatments are available, they increase the risk of complications and often have poor adherence. Aaron and his team wanted to find a solution that could directly target the injured system with high efficacy and negligible side effects.
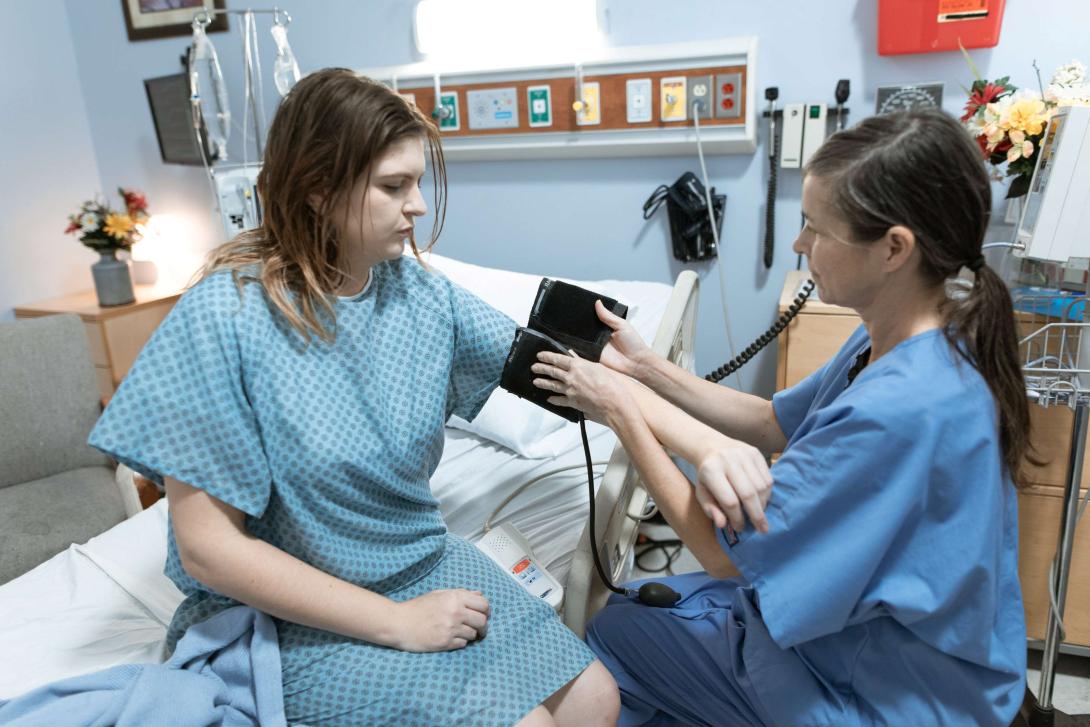
Developing new approaches for solving blood pressure instability after spinal cord injury has been the focus of the Phillips Lab for the past five years.
A pre-clinical model for hemodynamic instability
When developing a treatment for hemodynamic instability, the Phillips Lab ensured they deeply understood the sympathetic nervous system's involvement.
“My philosophy is that it's critical to understand the mechanism of the biology before you try and develop something new,” Aaron says. “I think it's very challenging, and perhaps impossible, to understand how best to optimize the intervention without a very strong understanding of the underlying biological mechanisms.”
The first preclinical model explored by Squair et al. was a tyrosine hydroxylase (TH)-Cre rat with a clinically-relevant contusion to the upper thoracic spinal cord (T3). The Phillips group visualized the damage to the descending pathways that regulate hemodynamics. By virally injecting neurons with genetically expressed fluorescent labels, neurons projecting down the spinal cord from the rostral ventrolateral medulla (RVLM) could be labeled. This is important as the RVLM is the region of the brain responsible for activating the sympathetic system’s control of cardiovascular function. This allowed Aaron and his team to track the baroreflex’s sympathetic pathway from the brain through the spinal cord.
This model revealed a near complete reduction of sympatho-excitatory synapses onto neurons below the injury.
In order to characterize how this lack of sympathetic cardiovascular control impacted the hemodynamics of the rat model, the Phillips group implanted Kaha SNA and Pressure Telemeters for long-term and concurrent measurement of sympathetic renal nerve activity and arterial blood pressure.
“It was a terrific tool,” Aaron says. “Firstly, it provided us with the necessary data to have a deep insight into the way the cardiovascular and sympathetic nervous systems were behaving after a spinal cord injury over time in the daily living of freely moving animals. It allowed us to understand how those systems responded to perturbations over time.”
“That insight gave us the evidence to determine that the rats were resistant to orthostatic challenges, which was crucial information in characterizing the model.”
“It also allowed us to understand how the sympathetic and cardiovascular systems were interacting.”
The Phillips group developed a completely novel preclinical negative pressure chamber below the mid-abdomen, pulling the blood toward the hindlimbs of the animal. This new device allowed for orthostatic hypotension to be studied in preclinical models of spinal cord injury for the first time. The Kaha Telemeter confirmed that this orthostatic challenge resulted in the same hemodynamic changes observed in a clinical ‘tilt-test’ in humans with spinal cord injury.
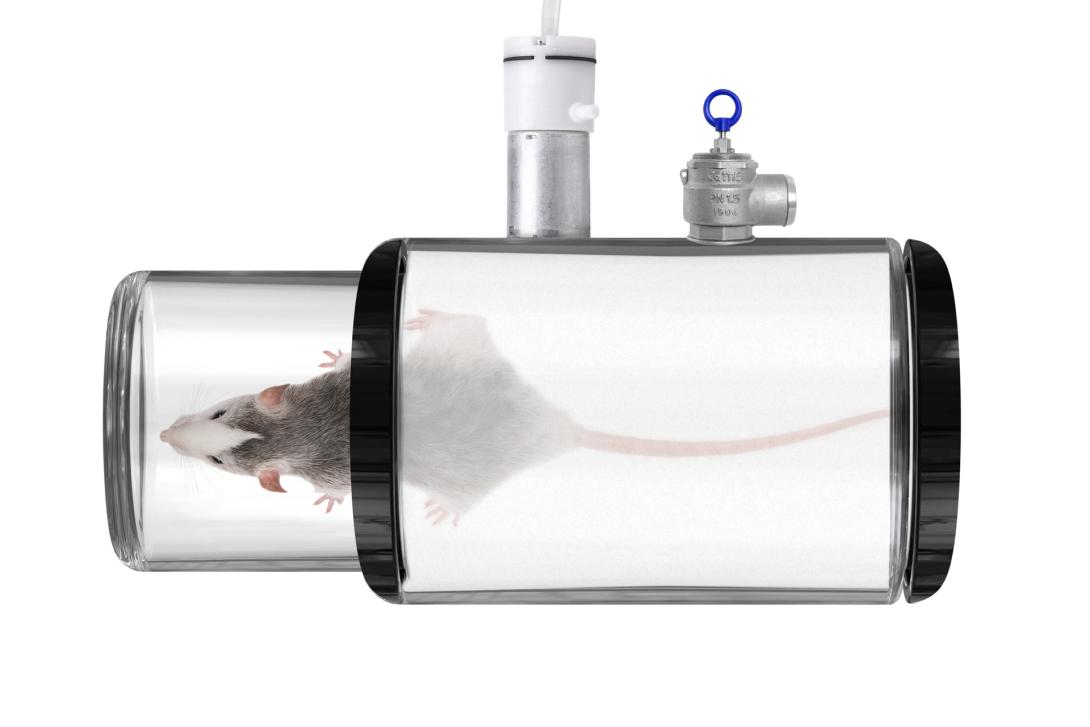
Uninjured rats experienced transient hypotension before a quick recovery, while rats with a spinal lesion experienced sustained hypotension linearly correlated with the chamber's pressure.
Stimulating hemodynamic hotspots
The team employed retrograde tracing from the splanchnic sympathetic ganglia - a collection of ganglionic fibers that house neurons projecting to blood vessels involved in blood pressure regulation - to identify optimal targets for stimulation. The retrograde tracing of these fibers revealed peak concentrations of spinal cord neurons involved in blood pressure control in the low thoracic region, around T12.
When epidural stimulation to the posterior roots (TESS) was applied to each spinal segment, Kaha Telemetry showed increased blood pressure during stimulation to T11-13. These hemodynamic hotspots correlated with neuron density from the retrograde tracing. It was determined that TESS applied to T11-13 primarily recruits large-diameter afferent fibers in the posterior roots to execute this pressor response. This was confirmed following the observation that the ablation of these afferent projections correlated to pressor response suppression during TESS.
“None of the steps involved in this work were trivial, but the viral tracing was among the most difficult aspects, particularly within the spinal cord,” Aaron says. “We spent a lot of time on trial and error, finding approaches so that we could understand the interneuronal activity necessary to have blood pressure and the sympathetic nervous system activate in response to epidural stimulation. We spent a lot of time and resources on that.”
Stimulation that mimics reality
To provide a long-term solution for hemodynamic instability after spinal cord injury, Aaron and the team began trialing protocols to mimic normal sympathetic nervous activity, replicating this activity below the site of spinal cord injury. The Kaha SNA and Pressure Telemeters were a crucial part of this testing, providing constant real-time information about sympathetic nerve and hemodynamic activity as trials progressed.
“It is so important to not just be able to measure these types of things in a single acute preparation where the animal is terminated after,” Aaron says. “It’s critical to characterize how things are occurring and progressing over time with any sort of injury or intervention. It’s the best way to identify whether something is getting worse or getting better. There was nothing else on the market that permits these concurrent approaches. We’ve been using them for years now.”
An electronic dura mater (e-dura) stimulator was fabricated and implanted against the spine to target the posterior roots of the T11-13 spinal segments, the hemodynamic hotspots. Recording activity from neurons in the rostral ventrolateral medulla showed the TESS protocols effectively mimicked the spatial sequences, frequency contents, and temporal profiles underlying natural sympathetic circuit activation. Using epidural stimulation, Aaron and the team created a neuroprosthetic baroreflex capable of stabilizing previously unstable hemodynamics in the acute phase of injury when neurogenic shock is occurring, and during orthostatic manoeuvres.
The first step toward translation
E-dura implants were scaled to suit the anatomy of non-human primates. Following acute, complete, spinal cord injuries at T3, their pressor responses to TESS were mapped. As with the rodents, hemodynamic hotspots were present in the lower thoracic spinal cord. An immediate spike in pressure post-injury, followed by profound hypotension, replicated patterns observed in humans.
Continuous TESS was able to temporarily rescue blood pressure, however, this ceased within a few heartbeats. Utilizing a clinical-grade wireless implantable pulse generator, the Phillips group were able to adapt their closed-loop system to align with the physiological data of the non-human primates. Once calibrated, the neuroprosthetic baroreflex instantly normalized blood pressure and stabilized the previously unstable hemodynamics of their subjects, independent of neurogenic shock and orthostatic challenges.
Translational research to benefit patient lives
In the final stage of their research, the Phillips group investigated the efficacy of their treatment in a human with chronic, functionally complete, cervical spinal cord injury. As a result of their injury, the patient experienced debilitating orthostatic hypotension. There were clear benefits if the treatment did prove effective in humans.
A paddle electrode array was surgically implanted below T10 and T11 to best target the hemodynamic hotspots identified in preclinical models. TESS induced robust pressor responses and normalized circulating levels of norepinephrine and increased sympathetic nerve activity, confirming the activation of sympathetic nerve circuitry. When challenged with a ‘tilt-table’, a simulated closed-loop titration of TESS amplitude was all that was needed to counteract the orthostatic stress and maintain hemodynamic stability.
With the long-term implementation of TESS, the patient was able to end medical treatments for hemodynamic instability, participate in vertical motor rehabilitation, and completely resolve orthostatic hypotension.
“In research, you’re often gathering huge amounts of data, and you don’t get to see whether or not your experiments have worked until it’s all been analyzed and shown to be statistically significant,” Aaron says, “But this just works - you can see it with your naked eye when you turn it on. It stabilizes blood pressure every time, it works across species, it works in the acute and chronic setting; it just works.”
So, what’s next? For the neuroprosthetic baroreflex, the journey has just begun. It’s barreling toward clinical studies and being done around the world. “It’s super rewarding to be able to discover something so robust,” Aaron says. “There were some surgeries on patients in Switzerland with the Courtine group that we collaborated with on the paper. I was able to attend these in person, and it just keeps working and working. We were all high-fiving in the operating room.”
“I don't want to overstate it by any means, but it is having a big impact. We're working with a publically traded company called ONWARD MEDICAL that is fully committed to bringing this therapy to the community through pivotal clinical trials, which we've licensed our patents to, and they've partnered with groups like the Christopher and Dana Reeves Foundation and the US Military (DARPA),” Aaron says, “There's a rapid amount of energy and acceleration in this space to bring the therapy to the community.”
“The reality is that the key steps to get this to the community are happening now. We have implanted two additional patients in Calgary, and we are validating the surgical approach and responses. Several more have taken place in Switzerland with Dr. Courtine. ONWARD received the FDA breakthrough designation for the approach. We're doing this now. The train has left the station.”
For more information, see these articles published in Nature:
Squair, Jordan W., et al. “Neuroprosthetic Baroreflex Controls Haemodynamics after Spinal Cord Injury.” Nature, vol. 590, no. 7845, 2021, pp. 308–14. https://doi.org/10.1038/s41586-020-03180-w
Soriano, Jan Elaine, et al. “Longitudinal Interrogation of Sympathetic Neural Circuits and Hemodynamics in Preclinical Models.” Nature Protocols, vol. 18, no. 2, Nature Portfolio, Nov. 2022, pp. 340–73. https://doi.org/10.1038/s41596-022-00764-w
Additional Resources
Translational Science Panel: Research Insights and Best Practice for Clinical Relevance »
Next-Generation Techniques for Understanding Neural Hemodynamic Control »
Four Cardiovascular Applications Using Kaha Sciences Telemetry »
Five Unique Neuroscience Applications Using Telemetry »
Rat Telemeter Product Range Overview – Kaha Sciences »
SmartPad Product Features – Kaha Sciences »
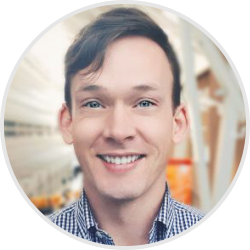
Aaron Phillips, Ph.D.
Dr. Aaron Phillips is an Associate Professor at the University of Calgary. Driven by his passion for the complex interactions between the nervous and cardiovascular systems, including anomalous interactions contributing to clinical disorders, Aaron's research focuses on developing novel therapeutics for people with neurological health issues.